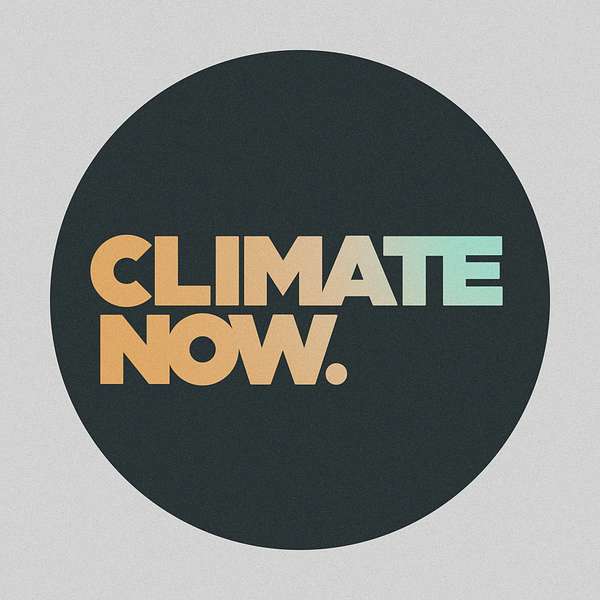
Climate Now
Explaining the key scientific ideas, technologies, and policies relevant to the global climate crisis. Visit climatenow.com for more information, video series, and events.
Climate Now
An interview with the scientist who achieved fusion ignition
Last week, LLNL's National Ignition Facility successfully 'ignited' a nuclear fusion reaction equivalent to what takes place in the sun: the conversion of hydrogen to helium + energy. In a first, the experiment produced more energy than was needed to initiate the reaction. While the experiment lasted only fractions of a second, it proved what had been hypothesized since the 1960’s: that lasers can be used to induce energy-generating fusion in a laboratory setting. The enormity of this achievement is that it brings the possibility of cheap, clean and safe nuclear fusion energy one step closer to reality. Joined by guest hosts Julio Friedman and Darren Hau, Climate Now sat down with Dr. Annie Kritcher, the principal designer for the successful fusion experiment, to discuss what they have accomplished, why it was so significant, and what the National Ignition Facility will be focusing on next in their work to make nuclear fusion a viable energy source.
Key Questions:
- What was the experiment that was performed, and why was it’s success so significant?
- What are the next set of challenges to address in developing nuclear fusion as a clean energy source?
Follow us on Twitter, LinkedIn, Facebook, and Instagram.
Contact us at contact@climatenow.com
Visit our website for all of our content and sources for each episode.
[00:00:00] James Lawler: Welcome to Climate Now, a podcast that explores and explains the ideas, technologies and on the ground solutions that will need to address the global climate crisis and achieve a net zero future. I’m James Lawler, and if you like this episode, please leave us a review wherever you get your podcasts, share this episode with your friends, or tell us what you think at contact@climatenow.com. We love hearing from our listeners.
Today’s episode features a special guest from a very exciting recent scientific breakthrough: Dr. Annie Kritcher is a physicist and the principal designer for the fusion experiment that reached ignition recently at the Lawrence Livermore National Laboratory.
But before we dive into that, let’s start with our news segment: This Week in Climate News. To talk through the news this week I’m joined by previous Climate Now guest, Dr. Julio Friedmann, who is Chief Scientist and Head Carbon Wrangler – what a fantastic title – at Carbon Direct. Julio, welcome.
[00:00:56] Julio Friedmann: My pleasure to be here.
[00:00:58] James Lawler: Our actual interview is with the major story of the last week or two, and we’ll get to that. But there are two other stories that we wanted to, to highlight. Julio, do you wanna jump in first?
[00:01:07] Julio Friedmann: Sure. So the first one is actually the California Public Utilities Commission, also known as the CPUC, has revised its rules around rooftop solar incentives and subsidies, specifically in the context of net metering rules. And this is a big change because it’s really going to alter the economics for people who install solar on their own home.
[00:01:32] James Lawler: And prior to this rule, the complaint had been from utilities that they were having to pay too much for electricity flowing back onto the grid from residential solar.
[00:01:40] And that that pricing should not be the same as retail pricing. And that’s been the debate. Right?
[00:01:45] Julio Friedmann: Right. I found it noteworthy that the decision for this was unanimous. All five commissioners agreed that this change was necessary.
[00:01:54] James Lawler: The other interesting announcement this week was that a team in China has developed an electrolysis process producing hydrogen from seawater, which is first of its kind in terms of the approach to use saltwater directly as a source of hydrogen.
And the process appears to do this by combining a desalination step and an electrolysis step within the same fuel cell. Julio do, do you want to jump in on this in terms of, you know, how this is achieved and, and how, how important this.
[00:02:21] Julio Friedmann: Yeah, absolutely. So first of all, it’s not the first, it’s just the first like real scale.
So a lot of this stuff has been done in laboratories, but these guys operated for like 130 hours, or I think it was actually generated 130 tons of hydrogen or something like that. Ran for a long time and in a commercial context. So that’s sort of the big breakthrough. And they were able to do this at what looks like reasonable costs.
So it is in fact membrane distillation combined with alkaline electrolysis. Alkaline electrolysis is the workhorse of hydrogen production by electrolysis today. The other being proton exchange membranes, or PEMs, and so there is a small advantage that you get from energy doing such a thing, by producing hydrogen this way, and so there’s potentially some Op-Ex savings because you’ll have a overall efficiency.
It is not clear whether it will actually be cheaper. Let’s start with that cuz the capital expenses may be higher. It is also the fact that [00:03:20] desalination technology is pretty cheap. Commercial desal only adds something like 10 cents per kilogram to the price of hydrogen. So it’s not a colossal breakthrough in that context.
I think it’s noteworthy. There will probably be some markets where this has an advantage because of the episodicity of the supply or the true lack of availability of freshwater, but fundamentally, this technical breakthrough is interesting. Could be locally useful, but I don’t consider it to be a big commercial breakthrough at all.
I consider it to be just another tool in the way that we can do electrolysis.
[00:03:57] James Lawler: As I mentioned earlier, Dr. Annie Kritcher is a physicist and the principal designer for the fusion experiment that reached ignition recently at the Lawrence Livermore National Laboratory. Joining me in the interview with Dr. Kritcher are Climate Now guest hosts Julio Friedmann and Darren Hau. And Julio Friedmann actually worked previously at Livermore National Lab within the National Ignition Facility, so we’re excited to share some of his insights along the way.
Well, Annie, I, I’d love to start just by understanding you know exactly what was achieved at LLNL and if you could just describe what the experiment was and what the outcome was.
Yeah,
[00:04:35] Annie Kritcher: Yeah, sure. So first I’ll talk about the experiment. So what we do is we have 192 giant laser beams. It’s the the largest laser in the world. We take those laser beams and shine them onto the inside of a gold can, or what we call a hohlraum. We create a really intense x-ray radiation bath, about 3 million degrees.
That bath irradiates the outside of a [00:05:00] BB-size capsule that contains our fusion fuel. That causes the outside of the capsule to explode outwardly, and that sends the fuel inwardly in a rocket-like effect. And this gives us the ability to make conditions that are more than two times the pressure of the center of the sun and about five times as hot as the center of the sun.
So when we do that and we make those conditions, we can actually be self-sustaining in the plasma for a given amount of time. So that means the heating from the fusion itself is outweighing the losses, the energy losses in the system, and that essentially helps us get to higher yields and higher fuel burnout fractions.
So the recent experiment made a few changes or design changes to build on an experiment from August of 2021, and this let us burn up about a factor of two more times of the fuel than was done previously. And what this ended up giving us in terms of fusion energy output is more than the laser energy that’s incident on the target to drive the implosion.
And then, kind of an interesting tidbit. So we get all that energy in about 90 trillionths of a second. So if you look at powers versus power-on-target, it’s, it’s really quite amazing. It’s about 30 quadrillion watts.
[00:06:16] James Lawler: Wow.
[00:06:17] Annie Kritcher: But for 90 trillionths of a second.
[00:06:20] James Lawler: Can you articulate or kind of frame the significance of this particular step forward?
It obviously has built on a number of experiments and results to date, but, was it unexpected that this would be sort of achievable or what were the expectations going in?
[00:06:35] Annie Kritcher: Sure. So I guess there’s kind of three par parts to that. How did we build on the [00:06:40] prior understanding, what is a significance, and did we expect to get that performance?
So I mean, ever since the commissioning of NIF we have been trying to continuously improve the plasma parameters to be able to get to the ignition condition and also then start to burn more of the fuel. So each step has led to some understanding that has fed into a new design. And the design that reached ignition here, gain of one, is called Hybrid E, and it’s a design where we’ve been optimizing things to try to make the implosion larger and that couples more of the driver energy to the hot plasma.
And it takes a while to sort of get the design details right, and also the experimental details like the target quality. That’s been a huge R&D effort over the last few years.
[00:07:28] James Lawler: What do you mean by target? What is target quality?
[00:07:30] Annie Kritcher: Yeah, good question. So we have the hohlraum where we shine the lasers into the hohlraum, and then inside the hohlraum sits the capsule that holds the fusion fuel and that capsule is made out of, in these experiments, it’s made out of high density carbon.
So amorphous carbon. And the way that that’s fabricated with very little defects has been continuously improved over the last several years. So there’s, it’s really a culmination of a lot of improvements over the years to come together. We had the August experiment in 2021, which got 1.35 megajoules of fusion yield.
So the idea here was how do we get higher and, and what do we have to do? We did have quite a bit of trouble with the capsule quality following that experiment. So that experiment used like the best capsule we’ve ever shot on NIF, the [00:08:20] highest quality capsule. And so the idea was can we make more design changes so we’re not as sensitive to.
You know, to the fielding condition so we don’t have to use perfect capsules that are really hard to make? And so that’s this new effort and it’s only been two shots so far. And so what we did is make the capsule a little bit thicker. And just in doing that, that helps us burn – it holds the implosion together longer to burn more of the fusion fuel. But we also have more margin for getting ignition in the first place, even when we have target defects.
And so going from, the first experiment in this campaign was in September, and then going into this one we made a symmetry correction to make the implosion more symmetric based on some recent experimental data, and all of, we had three separate pre-shot predictions, all of which said, as long as nothing goes catastrophically wrong, like a laser misfire or a particle falling on the capsule, they all predicted about a factor of two, two and a half increase in performance, which is what, what we saw. I think the, the surprising thing is whenever you’re extrapolating your models and your designs out to a space that we’ve never been to before, um, there’s always some uncertainty as to whether or not we can actually get there. But we did have the pre-shot predictions that indicated that was possible.
[00:09:34] Annie Kritcher: But I think what this means is, this is sort of the start. We, we are by no means ready to plug NIF into the grid. I think that was a great way that Kim Badillo said that. It’s a basic research facility. It’s not meant to generate electricity for the grid, but I do believe it’s the first step in a long, long road of development that will need to happen along many aspects and lasers even more design [00:10:00] developments, higher gain targets, and other technology developments, and obviously at the same time, we’re already using these experiments to support the nation.
[00:10:10] Darren Hau: Annie, you alluded to this already, but could you briefly describe the difference between wall plug energy and in this case having ignition fusion versus net energy gain?
[00:10:20] Annie Kritcher: Yeah, so I think when people say net energy gain, that’s confusing. We’ve been trying to refer to it as target gain. So we need… the wall plug energy is what’s used to generate the laser energy that we put on target, so the amplifiers, so that’s about 326 megajoules. And then once we get all the way down to the target and, and the laser conversion into 350 nanometer light, and it’s about two megajoules of laser energy, the on the actual target that we’re shooting, and about three megajoules of fusion energy out.
[00:11:00] Darren Hau: So just to reiterate, 300 megajewels from the grid gets you two megajoules from the lasers, which then gets you three megajoules coming out from the target.
[00:11:09] Annie Kritcher: That’s correct.
[00:11:10] Darren Hau: So clearly, as you mentioned, still a lot of work to happen. One question I had was, you know, fusion seems to be still the purview of advanced government labs like yours.
are there areas that maybe private industry could work on from that 300 megajoules to two megajoules that could be helpful for your effort?
[00:11:28] Julio Friedmann: So if, if I can weigh in here a little bit. This is actually something I know a little bit about. Let’s start with the fact that NIF was designed in the eighties.
They began construction in the nineties, right? This is basically a 40 year [00:11:40] old facility. There has been huge advances in lasers. In super capacitors and all these sorts of things. So is there a way to get it done? It’s already developed, actually. There are many things that can be done in that facility to improve all of those things.
And the design criteria of the time were, again, for a particular function and purpose, which was not necessarily an energy function and purpose. And the team at NIF over the years has in fact come up with conceptual designs for what an energy project would look like. It looks radically different in size and scope and scale than the kind of thing that facility that NIF is today.
So that work, unsurprisingly, is already underway. It is also the case that building NIF has delivered a set of commercial innovations to the market. Things like rolled glass, things like crystal construction. These have already gone off into the market and and created value in the economy. And to your last point Darren, there is of course a lot of commercial investment now in the fusion space. There’s $5 billion that have gone to commercial investment. Most of that is not inertial confinement fusion, the kind of laser based thing that Annie has talked about. Instead these are the donut shaped torroidal systems and stellarators and things like that for magnetic confinement fusion.
But the learnings from the plasma physics actually go back and forth between both and the, some of the discoveries that have come out of NIF have advised the magnetic confinement fusion community and vice versa. Actually, things that has been happening in the commercial sphere and in other research laboratories, say around ITER and so forth, have come back and advised the way that NIF does its work.
So for me, I, I sort of take all of this news in a very different kind of context. I’ve seen a lot of technologies [00:13:20] stumble and people are hypothetical about whether or not we can do it, and then they go from zero to one, and they show that the core thing that you need to do is doable. And at that point, immediately people start throwing rocks at it and they’re like, oh, well that’s, that is a stupid way to do a stupid thing.
And we’re like, yes, cuz that’s not what it was for. This was to show that we could go from zero to one. And so now it’s sort of off to the races. Now you’re haggling over price, you’re haggling over design. It’s a different conceptual landscape because you have done the thing that was impossible before that. You’ve shown that you can get gain.
I would say, of course there is a long way to go before a commercial reactor of any kind, and some of that is still gonna happen in NIF. They got a factor of, you know, 50% gain. They’ve gone from basically two megajoules to three megajoules. Awesome. You need to get more like a factor of 20 or a hundred gain. With the existing system that’s gonna be tough because it wasn’t built for that. Again, it was built for something else, but we actually need to get much higher gain out of this system. We also then need to have much more rapid target delivery.
[00:14:24] James Lawler: And when you say target delivery, what does that mean?
[00:14:26] Julio Friedmann: So Annie was talking about these hohlraums, these little capsules that they put in. Right now, the, when they’re banging on all cylinders, NIF can do about three of those a day. It is more common to do one of those a day. To get to a commercial reactor, you need to do like 10 a second, so wildly more, right? That also means they can’t be made out of gold and perfect precision. You’re gonna have to make huge volumes of these things very quickly, and you also have to manufacture the tridium and deuterium that go into them.
So all of that manufacturing and supply chain has to be scaled up If this is gonna be commercial at any point. The fact that these are real [00:15:00] manufacturing and commercial challenges shouldn’t be underestimated, but it is also the case that they are not the science challenges. There’s a parallel set of science challenges as well.
But all of that enters the realm of, okay, well how do we go from here to there? Then, now that we know that we can do this bit of fusion? How do we actually try to make it useful? We can talk about it in the context of climate change later, but I don’t wanna underestimate how huge a deal this is. It is a huge, huge, huge step forward despite all of its commercial limitations.
[00:15:27] James Lawler: Well, Annie, that’s a good pivot back to you. I mean, where will NIF focus, now that this result has been achieved?
[00:15:33] Annie Kritcher: Yeah, I mean, it’s a great question because a lab is huge also, and other than my specific area, there’s many of these efforts that are moving forward. So specifically on the design side, we’re looking at, we are trying to push up the yield even more.
We’re looking at maybe 10 megajoules without a laser upgrade and upgrading the laser just a little bit can maybe even let us get to the 20 megajoule level. And we’re talking about that’s with 2.6 megajoules in, so that would increase the gains quite a bit. We have people, projects and researchers looking into 3D printing these capsules so that we can mass produce ’em at a much cheaper rate to the quality that we need.
We have a machine learning team who’s gonna look at how to analyze the data and reoptimize the system on a fast time scale. We have the whole laser team. They maintain the laser and they do the upgrades, but they also look at new technologies. As Julio said, so many new technologies have already come out of the lab and they’re still working on more.
So we’re trying to advance on, on many different fronts, and there’s teams that are looking at fast target delivery systems, [00:16:40] but in my focus area it’s, you know, how do we get more out?
[00:16:43] James Lawler: How do you increase that ratio of, of energy at the target to megajewels released?
[00:16:48] Annie Kritcher: Yeah, I mean, so the, the thing that we just did, making the capsule thicker, it helps the implosion on so many different fronts.
One of the issues we have now is the implosion explodes too fast.
[00:16:59] James Lawler: When you say the implosion happens too fast, where, what do you mean? So yeah, the, we’re imagining lasers, a bunch of lasers focus on, it’s very small target.
[00:17:07] Annie Kritcher: Okay. So the lasers make an x-ray bath, which then heats and –
[00:17:13] James Lawler: What is an x-ray bath?
[00:17:14] Annie Kritcher: What is an x-ray bath?
Where do we even start?
[00:17:17] Julio Friedmann: To try to simplify, you’re turning one kind of light energy into another kind of light energy. It actually starts as infrared, then goes to ultraviolet, and then hits the hohlraum, and then that converts into x-rays, which is another kind of ultraviolet radiation, and that that’s what creates the heat and pressure.
It’s what’s called indirect drive. So the lasers aren’t actually pointing at the tridium, they’re pointing at something else, and that something else is what drives the reaction. Back to you, Annie.
[00:17:42] Annie Kritcher: Yeah, thank you. And so it’s a little x-ray oven and that heats the outside of the capsule and that makes that material basically explode out, kind of like rocket fuel. That sends the fuel inward at extreme velocities.
That’s the implosion phase. When the fuel is at its smallest volume, that’s when you get most of the neutrons are being produced, and we’re competing with the amount of heating we’re getting from the reactions, helping to heat the plasma to the losses. There’s energy losses in the system. There’s three main ones.
We radiate the energy away. We conduct the energy away, but we also, due to its [00:18:20] own pressure, because it’s at extreme pressures, it explodes back out. And that cools everything down really fast. And so it’s basically a race against this cooling. And so the idea is if we can keep the assembly together longer, we can sit there for longer and burn up more of the the fusion fuels.
So at this point in time, we’ve only burned about 4% of the fuel in the system that we have. And so even from the system we have with some design improvements, we could get quite a bit more fusion energy out. So there’s that. There’s also scaling the implosion larger with a little bit more laser energy, and that helps you absorb more of the driver energy, larger fraction of the driver energy.
And we’re continuing to work on the capsule quality. That, those are kind of the main directions that we’re pushing in, which will help us get to higher fusion energy out versus not that much extra laser energy in. So we kind of made the most difficult problem on ourselves here trying to, we’re trying to implode a very thin shell and you have these hydrodynamic instabilities and we’re trying to get just enough in to get it to ignite and hold it together for just long enough so everything’s sort of teetering on this edge of, it has to be perfect on all fronts.
Getting a little bit more driver energy in relaxes many of those conditions to a degree that’s gonna be you know, more cliff like than linear.
[00:19:41] Julio Friedmann: The thing to understand in this is, is that it’s a really tiny Goldilocks zone. You need to get everything just right. And so a lot of the simulation, a lot of the plasma physics work, a lot of the AI is really aimed at sort of tuning.
They have the core [00:20:00] materials, but there’s a lot of parameters to tune. Everything from the shape of the laser pulse to the size of the capsule, all these sorts of things. And so this campaign that they’re banging through is just checking every box in the matrix. We gotta go through every one of these things.
And then when they learn something, they’re like, ok, we need new matrix. Maybe we should have been the old one to do the new one. So understanding how small the goldilock zone and how many parameters there are is part of the reason why it’s taken so long. It’s part of the reason why it’s proper science.
It’s, it’s legitimately hard to do this, which is why 60 years of work have led to one example of gain.
[00:20:31] James Lawler: Could you tell us a little bit more about sort of the capsule and manufacturing the capsule? First, how, how big is a capsule actually and, and what does it take to produce one currently? Does it happen at the lab, does it happen somewhere else? How might this be accelerated?
[00:20:46] Annie Kritcher: Yeah, it’s a super R and D effort right now, and as I walk you through it, you’ll think of, you know, there’s many ways to speed this up or streamline this. The capsule batches, they come in batches of about 30, and you can work your way through coding one of these batches of capsules in like a few weeks, but there’s like, there’s different steps to polishing them. So, you know, relaxing the, the design requirements to how much you need to polish the surfaces back. That will save quite a bit of time. Between when you start coding and all the polishing steps and getting it into a target configuration is many months.
But it can be a continuous line of generating these targets. These targets, right now, they’re fabricated at a specialist facility in Germany called Diamond Materials, but we have gotten that technology here now at Lawrence Livermore National Laboratory, and we’re running the same coders. So what we do now is we have chemical vapor deposition.
[00:21:40] We deposit diamond onto the outside of mandrels, of silicon mandrels. And then we take those capsules that are created, ship them to General Atomics, drill a small fill tube. This is the tube that’s in the side of the capsule, and that’s how you get the fuel in. It’s a two micron size tube, which is smaller than bacteria.
[00:22:02] James Lawler: Wow.
[00:22:02] Annie Kritcher: So it’s absolutely amazing that they can do this. Then they leach out all of the solid, uh, mandrel material out of this tiny little fill tube, and they’ve developed really fast ways of doing that over the last couple of years. And then that sort of gets metrologized and assembled into a target assembly here at Livermore.
So it does, the capsules, uh, from beginning to end are several months. You can kind of make 30 at a time in a batch and we’re continuously making batch upon batch. And several different institutions are involved at this point because it is such an R and D effort. And, and usually we pause in between batches to see if, is there a setting I can change to make the batch a little bit higher quality?
[00:22:48] James Lawler: How big is a, how big is one capsule?
[00:22:51] Annie Kritcher: It’s two millimeters about in diameter.
[00:22:54] Julio Friedmann: It’s like the size of a peppercorn. I just wanted to draw attention to something that Annie said in passing rather casually, like this “oh yeah and then this is the part where we do vapor phase deposition of diamond on the outside of this thing.”
Like people don’t understand like how sophisticated this process and this work is for vapor phase deposition. They gotta do a couple of different things. One of them is you have to put the material into vapor. You have to vaporize it so it’s a gas. When you’re talking about diamond that means you’re starting with carbon [00:23:20] and so you’ve got vapor phase carbon, right?
Then you have to control the temperature, pressure, and surface reactions to the point where what you’re depositing is one thing and not another thing. You don’t want it to accidentally deposit just carbon black or accidentally deposit charcoal or accidentally deposit graphite. You want to deposit diamond.
So you really have to, from this vapor phase with very, very small degrees of freedom, control the pressure and temperature and the surface kinetic of those reactions. So it’s just building a machine that does like this is. Not an easy thing to do, um, for these kinds of works. This is another example of, of sort of how the lab does its business.
Vapor phase deposition is now something that is used in many other industries for many other different kinds of applications, like making membranes, for example, something that is now done by vapor phase deposition. But doing vapor phase deposition of diamond is kind of an unusual thing.
[00:24:15] James Lawler: Why is that so important that these be coded with a diamond sort of coating?
[00:24:20] Annie Kritcher: Yeah. The, so the diamond blade or capsule, we have shot other materials like plastic and beryllium, so it doesn’t have to be diamond, but because the diamond is denaw, it has more mass, more density. You can make it thinner and still achieve the same rocket, effect the same, and achieve the same velocities that you could with something that’s not as dense.
And the reason that making it thinner is really important is because then it’s not a race to getting the laser beams in before the hohlraum fills up with plasma. So as you’re creating this X-ray radiation bath, you’re creating a plasma [00:25:00] filling effect of the hohlraum, but you’re still trying to get the laser beams to get into the hohlraum.
But as the plasma ingresses into the center of the hohlraum, that actually stops your laser beams from getting in. So when you have a thinner target, you can make the laser pulse duration much shorter in time, so you don’t need to get it in later in time when it could be clipped. And so that’s one of the main reasons that we, that we’re doing diamond here. A different facility with a different configuration, plastic is a great option also.
[00:25:32] James Lawler: So Annie or an Julio, I’m curious how each of you would answer this. Over the last few years, can you characterize the optimism or maybe pessimism and how that has evolved about our ability to achieve this outcome.
[00:25:46] Julio Friedmann: Yeah, so my, my quick take is knowing that something is inevitable doesn’t make it happen. Like this was e everyone reali, you know, stars do fusion. So everybody’s like, well, we know that we should be able to do this, but that’s not the same thing as actually being able to do it. This has led to the long-lived joke of, you know, fusion is the a future and it always will be, but be because, you know, you keep trying, you keep trying to keep trying to keep failing.
You learn as you go. And what I put that in part in the context of, of the name of your show Climate Now, because knowing that this was inevitable has not helped us in climate. It has not helped us reduce emissions and given where we’re at right now with the results from NIF and with the other commercial Tokamaks and Stellarators, we are still a long way off.
It is unlikely that this will substantially contribute in the near term to managing climate, and quite frankly, whether I’m optimistic or pessimistic is [00:26:40] beside the point, the work looks the same. We still gotta reduce emissions. We still gotta remove emissions. Like there’s a huge scope of work, which the IPCC has laid out.
That said, it’s not like 2050 is the end of time, right? like we know that there will continue to be energy needs. There will continue to be climate needs that happen after we get to a net zero world. And part of the thing that makes me excited about the results from NIF is that we are building to a future that is not only just inverdent, but where fusion energy creates and unleashes a whole new set of opportunities that are optimistic, rich and vibrant, and the fact that it’ll contribute to an opportunity for growth or opportunity for interstellar exploration. All kinds of things that fusion energy provides as an opportunity is, is part of what gets the team at Livermore up in the morning.
But part of what gets them up in the morning is just the, the banging of boards, like they’re like every day, they’re like, we got another experiment, we got another experiment. We’re gonna build an edifice of knowledge today. And they do. And that edifice of knowledge continues to feed the entire community of actors that are out there.
[00:27:45] James Lawler: Yeah, very inspiring. Thanks Julio. So Annie same question back to you.
So, yeah,
[00:27:50] Annie Kritcher: I mean, I guess there was a time when people were wondering, is the laser big enough actually, or do we need a bigger laser? And until you do something, you don’t know exactly what it’s gonna take. And like Julio said, you, you fix the things in front of you one at a time, trying to make progress day after day on the next shot, on the next shot, on the next shot.
You see something in your diagnostics, you fix it, and then at some point the hope. Or, [00:28:20] or the thought, is if you fix all the things, they should work like what we had thought. And they do. And that’s amazing. And right about back in October of 2020 is the first time we started to see ourselves coming out of the weeds, that we’re starting to fix enough of these things that, you know, we’re not killed by something else.
We we’re starting to make progress. And so, I think, and that wasn’t that long ago, a couple of years when we, you know, we had that boost of, okay, well, you know, it’s gonna happen now on NIF and, and then within each experiment a change to the next experiment. Like I mentioned, we always have our predictions and our, you know, what we hope to see, but sometimes you don’t see that and you have to figure out why, um, and try to fix it.
[00:29:05] James Lawler: Can you characterize the landscape of experiments and reactor types and kind of where would you, if I could could put it in this kind of crass way, where would you put your money in terms of the likely winners in this space?
[00:29:22] Annie Kritcher: Well, I’m not even allowed to answer that question, so.
[00:29:26] James Lawler: Fair enough.
[00:29:26] Julio Friedmann: Well, maybe I can take a whack at it then.
So there’s twos basic kinds of fusion. There’s, well, we’ve been talking about internal confinement fusion where you’re shooting lasers and causing a forced implosion and getting fusion that way. The other is magnetic confinement. These are, again, the donut shaped, or similar kind of shape. Donuts are called torroids. That’s the Tokomak, and a variant of that is a stellarator, which is a, a wacky design that physicists cooked up that is better and more efficient in some ways and harder than others. What you’re really asking is which miracle do you believe will come first? I don’t think we [00:30:00] are at a position where it makes sense to say I put my money on black as opposed to I put my money on red.
I think there’s a just a huge range of uncertainty out there. This begs the question, how should we spend our money? And frankly, I would like to see a whole lot more innovation going into all sides of this. Now that we have gained, it makes the case that we are no longer throwing money down a hole. It makes the case that we can actually get useful outta this.
Some of that useful is for the weapons program, understanding the weapons we make and use in building better deterrents. Some of that is science. Already NIF has revealed things like the physics of of Jupiter’s core, which is kinda kooky, but like we know stuff now about astrophysics that we did not know before from these kinda experiments.
And then at the last of course, people are like, Hey, can this heat my tea kettle? And the answer is someday. And how that will compete in a landscape of power, supplies, like nobody knows. I wonder actually whether or not any of this is really gonna be used for electricity generation. Cause we’re getting better and better at electricity generation with everything else.
Like it may be that nuclear fusion may never compete for electricity generation, but it may very well provide access to a whole new realm of energy applications we’re only beginning to think about. So if you’re, if you were to saying, which, where should I put my money for commercial power right now? Not gonna answer that question, just not. Premature. But if you’re saying, should we be putting money on these things, that’s a hell yes. And that’s where the government investments actually really reduce the risk, give benefits back to the public as a whole, reduce the capital shocks for investors, all these kinds of things.
One last thing I’ll say about this. [00:31:40] Now that the US has done this, we are gonna start seeing more countries diving into this space for real, because of the breakthrough that we’ve made here at NIF.
[00:31:47] James Lawler: Thanks Julio, that that was very helpful. Annie, do you have any thoughts on, on that?
[00:31:51] Annie Kritcher: My comment, and the national labs are gonna be collaborating equally with all the different new startup companies.
And so we’re, we’re not meant to indicate which one’s gonna work or not work. We’re meant to work with them and, and try to advance the science.
[00:32:08] Julio Friedmann: Most people don’t know this, the Energy Policy Act of 2009, the Department of Energy has to put a tiny fraction of every budget into commercialization, for real.
And Congress got serious about that a few years after they passed that law. And so the Department of Energy has a whole office, the Office of Technology Transitions, whose job it is, is to make discoveries that come out of labs and bring ’em to business and to foster collaborations of various kinds. So the fact that labs are getting better at this is also grounds for optimism.
[00:32:33] James Lawler: Well, Annie, tell me this, what was it like when you actually got this result? Was it a, was, was everyone like Yeah. Or was it. That’s what we thought.
[00:32:41] Annie Kritcher: No, I mean, I mean, we weren’t, none of us were together, um, because it happened at one in the morning, and so like nobody really goes onsite either anymore after Covid, except the people that are running the laser and setting up the diagnostics.
So I work with a lead experimentalist and so he sits up all night like manning the shot and, you know, I don’t, I’m on call, but I don’t have to be up all night. So he actually sent me a text message at 1:00 AM so that when I woke up, 3:00 AM , I would see that before, like anything else and just, you know, get super excited and no, it’s like everybody was, like I said, when you kind of are extrapolating [00:33:20] into a new regime, you have predictions, but until you actually see it happening, there’s always disbelief.
And so, um, we were ecstatic. It was like walking on air.
[00:33:32] James Lawler: That was Dr. Annie Kritcher, the principal designer for the fusion experiment that achieved ignition at the Lawrence Livermore National Lab. Thanks to this research, clean energy from fusion is closer than it has ever been before. That’s it for this episode of The Climate Now podcast. For more episodes, videos, or to sign up for our newsletter, please visit us at climatenow.com.
We hope you’ll join us for our next conversation!
Climate Now is made possible, in part, by our science partners, like the Livermore Lab Foundation. The Livermore Lab Foundation supports climate research and carbon cleanup initiatives at the Lawrence Livermore National Lab, which is a Department of Energy applied science and research facility. More information on the foundation’s climate work can be found at livermorelabfoundation.org.